Tag: CO2 emission
Nuclear Energy Renaissance: Powering Sweden’s Climate Policy
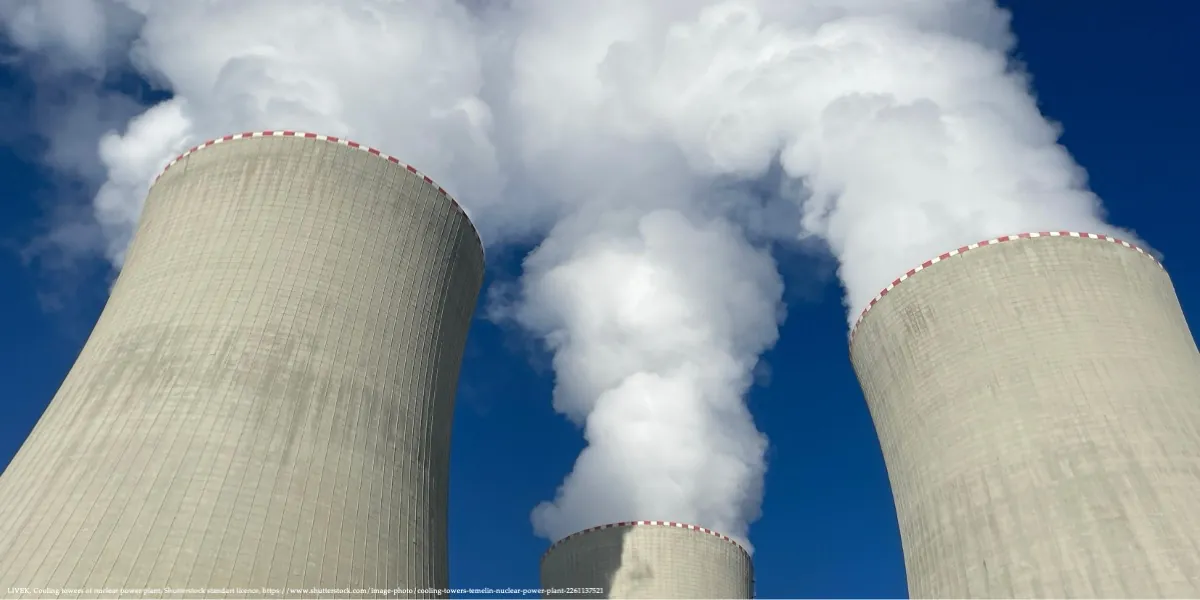
Sweden’s Nuclear Energy Expansion: Is It the Key to Net-Zero Emissions by 2045?
The Swedish government has placed nuclear energy at the forefront of its climate policies, aiming for two new reactors to be operational by 2035 and a total of ten new reactors by 2045. This policy brief explores whether the large-scale expansion of nuclear energy in Sweden is an environmentally and economically viable solution to achieve the nation’s goal of net-zero emissions by 2045. To assess this, we examine three critical factors: potential emission reductions, the cost-effectiveness of nuclear power, and the feasibility of the proposed construction timelines.
As a case study, we compare Sweden’s approach to nuclear energy with the successful nuclear build-out in France during the 1970s. France significantly reduced its carbon dioxide (CO2) emissions while reaping economic benefits, with an average reactor construction time of about six years. However, the situation in Sweden’s nuclear energy sector today differs from France in the 1970s. Sweden already has a low-carbon electricity grid, and the costs of alternative zero-carbon energy sources, such as wind and solar, have dropped considerably. Additionally, the construction costs and timelines for nuclear reactors in Sweden have increased compared to historical norms.
Thus, while nuclear energy in Sweden may contribute to modest emission reductions, the abatement costs are high, and reactor construction is expected to take much longer—up to two or three times longer than France’s build-out. This raises questions about whether Sweden’s nuclear expansion can effectively support the country’s ambitious climate goals.
A Renewed Focus on Nuclear Energy
When the current government in Sweden, led by Prime Minister Ulf Kristersson, came into power in 2022, they swiftly made changes to Sweden’s environment and climate policies. The Ministry of Environment was abolished, transport fuel taxes were reduced, and the energy policy objective was changed from “100 percent renewable” to “100 percent fossil free”, emphasizing that nuclear energy was now the cornerstone in the government’s goal of reaching net zero emissions (Government Office 2023, Swedish Government 2023). This marked a new turn in Sweden’s relationship with nuclear energy: from the construction of four different nuclear power plants in the 1970s – of which three remain operational today – to the national referendum on nuclear energy in 1980, where it was decided that no new nuclear reactors should be built and that existing reactors were to be phased-out by 2010 (Jasper 1990).
Today’s renewed focus on nuclear energy, especially as a climate mitigation policy tool is, however, not unique to Sweden. As of 2022, the European Commission labels nuclear reactor construction as a “green investment”, the US has included production tax credits for nuclear energy in their 2023 climate bill the Inflation Reduction Act, and France’s President Macron is pushing for a “nuclear renaissance” in his vision of a low-carbon future for Europe (Gröndahl 2022; Bistline, Mehrotra, and Wolfram 2023; Alderman 2022).
France As a Case Study
In the 1970s, France conducted an unprecedented expansion of nuclear energy, which offers valuable insights for Sweden’s contemporary nuclear ambitions. Relying heavily on imported oil for their energy needs, France enacted a drastic shift in energy policy following the 1973 oil crisis. In the subsequent decade, France ordered and began the construction of 51 new nuclear reactors. The new energy policy – dubbed the Messmer Plan – was summarized by the slogan: “All electric, all nuclear” (Hecht 2009).
To support the expansion of new reactors, the French government made use of loan guarantees and public financing (Jasper 1990). A similar strategy has recently been proposed by the Swedish government, with suggested loan guarantees of up to 400 billion kronor (around $40 billion) to support the construction of new reactors (Persson 2022).
France’s Emissions Reductions and Abatement Costs
To make causal estimates of the environmental and economic effects of France’s large-scale expansion of nuclear energy, we need a counterfactual to compare with. In a recent working paper – titled Industrial Policy and Decarbonization: The Case of Nuclear Energy in France – I, together with Jared Finnegan from University College London, construct this counterfactual as a weighted combination of suitable control countries. These countries resemble France’s economy and energy profile in the 1960s and early 1970s, however, they did not push for nuclear energy following the first oil crisis. Our weighted average comprises five European countries: Belgium, Austria, Switzerland, Portugal, and Germany, with falling weights in that same order.
Figure 1 depicts per capita emissions of CO2 from electricity and heat production in France and its counterfactual – ‘synthetic France’ – from 1960 to 2005. The large push for nuclear energy led to substantial emission reductions, an average reduction of 62 percent, or close to 1 metric ton of CO2 per capita, in the years after 1980.
Figure 1. CO2 emissions from electricity and heat in France and synthetic France, 1960-2005.

Andersson and Finnegan (2024).
Moreover, Figure 1 shows that six years elapsed from the energy policy change until emission reductions began. This time delay matches the average construction time of around six years (75 months on average) for the more than 50 reactors that were constructed in France following the announcement of the Messmer Plan in 1974.
Table 1. Data for abatement cost estimates.

Andersson and Finnegan (2024).
Lastly, these large and relatively swift emission reductions in France were achieved at a net economic gain. Table 1 lists the data used to compute the average abatement cost (AAC): the total expenses incurred for the new policy (relative to the counterfactual scenario), divided by the CO2 emissions reduction.
The net average abatement cost of -$20 per ton of CO2 is a result of the lower cost of electricity production (here represented by the levelized cost of electricity (LCOE)) of new nuclear energy during the time-period, compared to the main alternative, namely coal, – the primary energy source in counterfactual synthetic France. LCOE encompasses the complete range of expenses incurred over a power plant’s life cycle, from initial construction and operation to maintenance, fuel, decommissioning, and waste handling. Accurately calculated, LCOE provides a standardized metric for comparing the costs of energy production across different technologies, countries, and time periods (IEA 2015).
Abatement Costs and Timelines Today
Today, more than 50 years after the first oil crisis, many factors that made France’s expansion of nuclear energy a success are markedly different. For example, the cost of wind and solar energy – the other two prominent zero-carbon technologies – has plummeted (IEA 2020). Further, construction costs and timelines for new nuclear reactors in Europe have steadily increased since the 1970s (Lévêque 2015).
Figure 2 depicts the LCOE for the main electricity generating technologies between 2009 and 2023 (Bilicic and Scroggins 2023). The data is for the US, but the magnitudes and differences between technologies are similar in Europe. There are two important aspects of this figure. First, after having by far the highest levelized cost in 2009, the price of solar has dropped by more than 80 percent and is today, together with wind energy, the least-cost option. Second, the cost of nuclear has steadily increased, contrary to how technology cost typically evolves over time, meriting nuclear power the “a very strange beast” label (Lévêque, 2015, p. 44). By 2023, new nuclear power had the highest levelized cost of all energy technologies.
Regarding the construction time of nuclear reactors, these have steadily increased in both Europe and the US. The reactor Okiluoto 3 in Finland went into commercial operation last year but took 18 years to construct. Similarly, the reactor Flamanville 3 in France is still not finished, despite construction beginning 17 years ago. The reactors Hinkley Point C in the UK were initiated in 2016 and, after repeated delays, are projected to be ready for operation in 2027 at the earliest (Lawson 2022). Similarly, in the US, construction times have at least doubled since the first round of reactors were built. These lengthened constructions times are a consequence of stricter safety regulations and larger and more complex reactor designs (Lévêque, 2015). If these average construction times of 12-18 years are the new norm, Sweden will, in fact, not have two new reactors in place by 2035. Further, it would need to begin construction rather soon if the goal of having ten new reactors by 2045 is to be achieved.
Figure 2. Levelized Cost of Electricity, 2009-2023.

Source: Bilicic and Scroggins (2023).
Sweden’s Potential Emission Reductions
The rising costs and extended construction times for new reactors are notable concerns, yet the crucial measure of Sweden’s new climate policy is its capacity to reach net zero emissions across all sectors. Figure 3 depicts per capita emissions of CO2 from electricity and heat production in Sweden and OECD countries between 1960 and 2018.
Figure 3. Sweden vs. the OECD average.

Source: IEA (2022).
In 2018, the OECD’s per capita CO2 emissions from electricity and heat averaged slightly over 2 metric tons. In comparison, Sweden’s per capita emissions at 0.7 metric tons are low and represent only 20 percent of total per capita emissions. Hence, the potential for substantial emission cuts through nuclear expansion is limited. By contrast, Sweden’s transport sector, with CO2 emissions more than two times larger than the emissions from electricity and heat, presents a greater chance for impactful reductions. Yet, current policies of reduced transport fuel taxes are likely to increase emissions. The electrification of transportation could leverage the benefits of nuclear energy for climate mitigation, but broader policies are then needed to accelerate the adoption of electric vehicles.
Conclusion: Sweden’s Nuclear Energy Renaissance and Its Impact on Climate Policy
As Sweden rewrites its energy and climate policies, nuclear energy is placed front and center – a position it has not held since the 1970s. Yet, while nuclear energy may experience a renaissance in Sweden, it will not be the panacea for reaching net zero emissions the current government is hoping for. Expected emission reductions will be modest, abatement costs will be relatively high and, if recent European experiences are to be considered an indicator, the aspirational timelines are likely to be missed.
Considering these aspects, it’s imperative for Sweden to adopt a broader mix of climate policies to address sectors such as transportation – responsible for most of the country’s emissions. Pairing the nuclear ambitions with incentives for an accelerated electrification of transportation could enhance the prospects of achieving net zero emissions by 2045.
References
- Alderman, L. (2022). France Announces Major Nuclear Power Buildup. The New York Times. February 10, 2022.
- Andersson, J. and Finnegan, J. (2024). Industrial Policy and Decarbonization: The Case of Nuclear Energy in France. Working Paper.
- Bilicic, G. and Scroggins, S. (2023). 2023 Levelized Cost of Energy+. Lazard.
- Bistline, J., Mehrotra, N. and Wolfram, C. (2023). Economic Implications of the Climate Provisions of the Inflation Reduction Act. Tech. rep., National Bureau of Economic Research.
- Government Office. (2023). De första 100 dagarna: Samarbetsprojekt klimat och energi. Stockholm, January 25, 2023.
- Gröndahl, M-P. (2022). Thierry Breton: ’Il faudra investir 500 milliards d’euros dans les centrales nucléaires de nouvelle génération’. Le Journal du Dimanche January 09, 2022.
- Hecht, G. (2009). The Radiance of France: Nuclear Power and National Identity after World War II. MIT Press.
- IEA. (2015). Projected Costs of Generating Electricity: 2015 Edition. International Energy Agency. Paris.
- IEA. (2020). Projected Costs of Generating Electricity: 2020 Edition. International Energy Agency. Paris.
- IEA. (2022). Greenhouse Gas Emissions from Energy (2022 Edition). International Energy Agency. Paris.
- Jasper, J. M. (1990). Nuclear politics: Energy and the state in the United States, Sweden, and France, vol 1126. Princeton University Press.
- Lawson, A. (2022). Boss of Hinkley Point C blames pandemic disruption for 3bn delay. The Guardian. May 20, 2022.
- Lévêque, F. (2015). The economics and uncertainties of nuclear power. Cambridge University Press.
- Persson, I. (2022). Allt du behöver veta om ’Tidöavtalet. SVT Nyheter. 14 October, 2022.
- Swedish Government. (2023). Regeringens proposition 2023/24:28 Sänkning av reduktionsplikten för bensin och diesel. State Documents, Sweden. Stockholm, October 12, 2023.
Disclaimer: Opinions expressed in policy briefs and other publications are those of the authors; they do not necessarily reflect those of the FREE Network and its research institutes.
The Impact of Technological Innovations and Economic Growth on Carbon Dioxide Emissions
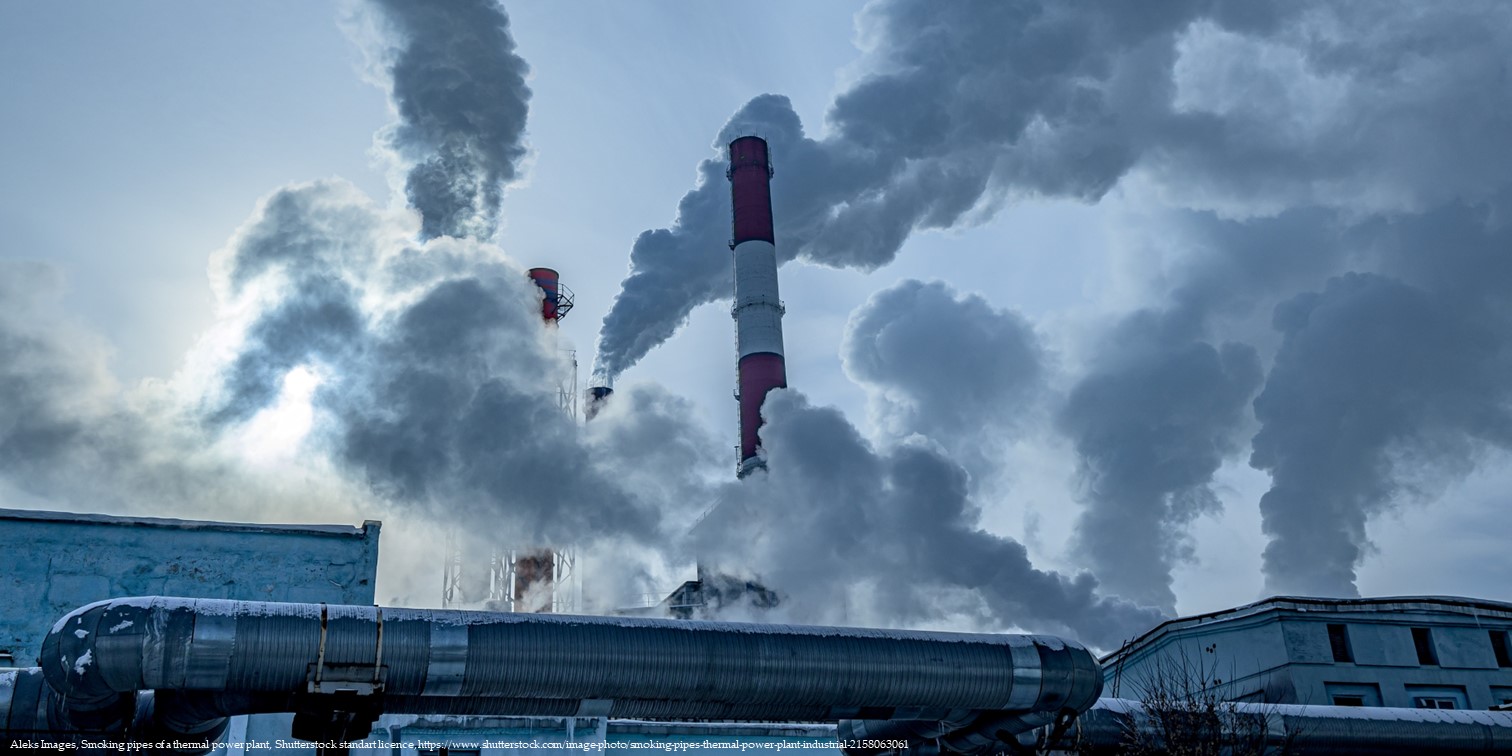
This policy brief offers an examination of the interplay between economic growth, research, and development (R&D), and CO2 emissions in different countries. Analysing data for 83 countries over three decades, our research reveals varying impacts of economic and R&D activities on CO2 emissions depending on country income level. While increased economic growth often leads to higher emissions due to greater industrial activity, our model indicates that increased GDP levels, when interacted with enhanced investments in R&D, is associated with reduced CO2 emissions. Our approach also recognizes the diverse economic conditions of countries, allowing for a more tailored understanding of how to tackle environmental challenges effectively.
Technological Innovation and CO2 Emissions
Human activity has over the past few decades significantly contributed to environmental problems, in particular CO2 emissions. The consequences from increased CO2 emissions, such as global warming and climate change, have motivated extensive research focused on understanding their impact and finding potential solutions to associated issues.
Economic growth, and research and development (R&D) can serve as differentiating factors between countries when it comes to their pollution levels, specifically measured by CO2 emissions per capita. Higher levels of economic growth are associated with increased industrial activity and energy consumption, which may lead to increased CO2 emissions. At the same time, countries that invest more in R&D often focus on developing cleaner technologies and implementing sustainable practices, which may result in reduced CO2 emissions.
In this policy brief, we analyse CO2 emissions’ dependencies on technological innovation and economic growth. For our analysis we group the considered 83 countries into three wealth levels: High, Upper Middle, and Lower Middle income levels. This grouping facilitates a better understanding of the complex interplay between wealth, innovation and growth and their projection into emissions. Considering each wealth level group separately also allows us to account for varying economic and developmental contexts.
Data
Based on data availability, we analyse 83 countries, spanning from 1996 to 2019, inclusive. We follow current research trends and use R&D intensity as a proxy for technological innovation (see Chen & Lee, 2020; Petrović & Lobanov, 2020; Avenyo & Tregenna, 2022).
Data on energy use originate from Our World in Data. R&D data from after 2014 are based on figures from the UNESCO Institute for Statistics. All other indicators come from World Development Indicators (WDI).
Table 1 presents an overview of the variables considered in our empirical model. Our response variable is CO2 emissions per capita. We include several covariates (i.e. urban population, renewable energy, trade), found to be significant in previous studies where CO2 emissions was considered the dependent variable (Avenyo & Tregenna, 2022; Wang, Zeng & Liu, 2019; Petrović & Lobanov, 2020; Chen & Lee, 2020).
Table 1. Variable description.
Additionally, we include quadratic terms for GDP and R&D to account for nonlinearity and non-monotonicity. Also, we incorporate the interaction term between GDP and R&D (see Table 3). This allows us to evaluate whether the impact of technological innovations on CO2 emissions is dependent on the GDP level, or vice versa.
Wealth Level Classification
Existing literature highlights significant variation between countries in terms of economic growth and income levels, particularly in relation to R&D expenditure and CO2 emission levels (see Cheng et al., 2021; Chen & Lee, 2020; Petrović & Lobanov, 2020; Avenyo & Tregenna, 2022). Given this we deployed the Mclust method (Scrucca et al., 2016; Fraley & Raftery, 2002), and classified our considered countries into three distinct groups based on their median Gross National Income (GNI) over a specified range of years for each country. Following this methodology, we obtained three groups of countries: High, Upper Middle and Lower Middle. The list of countries categorized by their respective wealth level is presented in Table 2.
Table 2. Countries within each wealth group.
Low-income countries, (as categorized by the World Bank in 2022) were not included in the analysis as the study focuses on the impact of technological innovations on CO2 emissions, innovations which are less frequent in such economies. Limited infrastructure, financial resources, and access to technology often result in lower levels of R&D activities in low-income countries, which reduces the number of measurable innovations.
The Hybrid Model
Our leading hypothesis is that country income levels (measured by GDP) mediates the relationship between innovation (measured by R&D expenditures) and CO2 emissions. To test this, one could estimate this relationship for each group of countries separately. This policy brief instead estimates the relationship for the whole sample of countries accounting for group differences via interaction effects. Specifically, our estimation allows for interaction terms between some or all covariates and the wealth level. This approach, which we refer to as the hybrid model, thus combines elements of both pooled and separate models. It is a great alternative to separate models as it allows for estimation of both group-specific and sample-wide effects, and as it contrasts differential impacts across wealth level groups.
We test two versions of the hybrid model, one full and one reduced. The full model incorporates interactions with all covariates while the reduced model includes some indices without interactions, resulting in a relationship shared across all wealth levels. The reduced model assumes that the variables Renewable energy consumption, Energy use and Trade exhibit the same relationship with CO2 emissions across all wealth levels.
Both the reduced and full hybrid models have similar coefficients for the variables and interactions that they share. While the coefficients share signs in both the full and reduced hybrid models, they are smaller, in absolute values, in the reduced hybrid model. In Table 3 we present the estimates from the reduced hybrid model.
Table 3. Results from the reduced hybrid model with CO2 emissions as dependent variable, by wealth group level.

Note: The upper part of the table (denoted “interaction variables”) depicts the coefficients for the interaction term between the variable in the respective row and the income group in the respective column. * denotes a 0.05 significance level. ** denotes a 0.01 significance level. ***denotes a 0.001 significance level.
Several things are to be noted from Table 3. First, for High and Upper Middle wealth level countries there is a significant positive association between innovation (as proxied by R&D) and CO2 emissions. However, the significance levels of the interaction term for R&D and GDP reveal that the relationship between R&D and CO2 is not constant across wealth levels even within each group. Specifically, it appears that relatively high values of GDP and R&D are associated with a decrease in CO2 emissions in High and Upper Middle wealth level countries. This suggests that in wealthier countries, advancements in technology and efficient practices derived from R&D are likely contributing to reduced emission levels. Interestingly, GDP has no direct effect on emissions for countries in these two wealth groups. Rather, GDP only affects emissions through the interaction term with R&D.
In turn, for the Lower Middle wealth level countries, R&D has no impact on CO2 emissions, whether directly or via interaction with GDP. Instead, higher GDP leads to a significant increase in emissions. This suggests that for these countries economic growth entail CO2 emissions while R&D activities are too small to have a mediating effect.
Second, medium and high-technology industry value added manufacturing is only significant for countries within the Upper Middle wealth level. This is in line with previous literature (see Avenyo & Tregenna, 2022, Wang, Zeng & Liu, 2019). A higher proportion of medium and high-technology industry value added is often negatively associated with CO2 emissions due to the adoption of cleaner and more environmentally sustainable technologies and practices within these industries. Additionally, these industries are often subject to stringent environmental regulations. As a result, these industries can contribute to reduced emission levels, becoming key drivers of sustainable economic growth and environmental protection (Avenyo & Tregenna, 2022). Interestingly, in our estimation, this result is evident only for Upper Middle wealth level countries.
Third, urban population is only significantly increasing emissions for High wealth level countries. Such positive relationship can be attributed to several factors. There is often a higher concentration of industrial and manufacturing activities in urban areas, leading to increased emissions of pollutants as urbanization increases (Wang, Zeng & Liu, 2019). Additionally, urban areas tend to have higher energy consumption and transportation demands, further contributing to higher emission levels.
When it comes to the factors jointly estimated across wealth groups, the positive relationship between renewable energy consumption and CO2 emissions is well-documented within the literature (Chen & Lee, 2020) which emphasizes the need for sustainable energy practices and efficient resource management to mitigate adverse environmental impacts. In line with this, the significant negative relationship between renewable energy consumption and CO2 emissions suggests that an increase in renewable energy usage is associated with a reduction in CO2 emissions. This is in line with previous findings demonstrating that technological progress helps reduce CO2 emissions by bringing energy efficiency (Akram et al., 2020; Sharif et al., 2019).
Conclusion
This policy brief analyses the effects of GDP and technological innovations on CO2 emissions. The theoretical channels linking economic development (and technological innovations) and CO2 emissions are multifaceted, warranting the need for an econometric assessment. We study 83 countries between 1996 and 2020 in a setting that allows us to disentangle the effects across countries with different income levels.
Our findings underscore the importance of considering the various income levels of the considered countries and their interplay with R&D expenditures in environmental policy discussions. Countries with Lower Middle income levels exhibit insignificant effects from R&D expenditures on CO2 emissions, while for Upper Middle and High wealth level nations, increased R&D expenditures incurs higher emissions.
The moderating role of GDP adds complexity to this relationship. At sufficiently high wealth levels, GDP weakens the effect of R&D on emissions. This alleviating effect becomes stronger as GDP increases until reaching a turning point, at which the impact reverses and R&D expenditures instead decrease emissions.
Our results on the significant nonlinear relationship between R&D, GDP and CO2 emission levels highlights the complexity of addressing environmental challenges within the context of macroeconomics. It suggests that policies promoting both R&D and economic growth simultaneously can foster more sustainable development paths, where economic expansion is accompanied by a more efficient and cleaner use of resources, leading to lower CO2 emissions. This decoupling of economic growth from emissions is likely to be further enhanced by governments incentivising research and development focused on improved energy efficiency and emission reduction.
References
- Akram, R., Chen, F., Khalid, F., Ye, Z., & Majeed, M. T. (2020). Heterogeneous effects of energy efficiency and renewable energy on carbon emissions: Evidence from developing countries. Journal of cleaner production, 247, 119122.
- Avenyo, E. K., & Tregenna, F. (2022). Greening manufacturing: Technology intensity and carbon dioxide emissions in developing countries. Applied energy, 324, 119726.
- Chen, Y., & Lee, C. C. (2020). Does technological innovation reduce CO2 emissions? Cross-country evidence. Journal of Cleaner Production, 263, 121550.
- Cheng, C., Ren, X., Dong, K., Dong, X., & Wang, Z. (2021). How does technological innovation mitigate CO2 emissions in OECD countries? Heterogeneous analysis using panel quantile regression. Journal of Environmental Management, 280, 111818.
- Fraley C. and Raftery A. E. (2002) Model-based clustering, discriminant analysis and density estimation. Journal of the American Statistical Association, 97/458, pp. 611-631.
- Petrović, P., & Lobanov, M. M. (2020). The impact of R&D expenditures on CO2 emissions: evidence from sixteen OECD countries. Journal of Cleaner Production, 248, 119187.
- Scrucca, L., Fop, M., Murphy, T. B., & Raftery, A. E. (2016). mclust 5: clustering, classification and density estimation using Gaussian finite mixture models. The R journal, 8(1), 289.
- Sharif, A., Raza, S. A., Ozturk, I., & Afshan, S. (2019). The dynamic relationship of renewable and nonrenewable energy consumption with carbon emission: a global study with the application of heterogeneous panel estimations. Renewable energy, 133, 685-691.
- Wang, S., Zeng, J., Liu, X., (2019). Examining the multiple impacts of technological progress on CO2 emissions in China: a panel quantile regression approach. Renew. Sustain. Energy Rev. 103, 140–150.
Disclaimer: Opinions expressed in policy briefs and other publications are those of the authors; they do not necessarily reflect those of the FREE Network and its research institutes.
Exploring the Impact from the Russian Gas Squeeze on the EU’s Greenhouse Gas Reduction Efforts
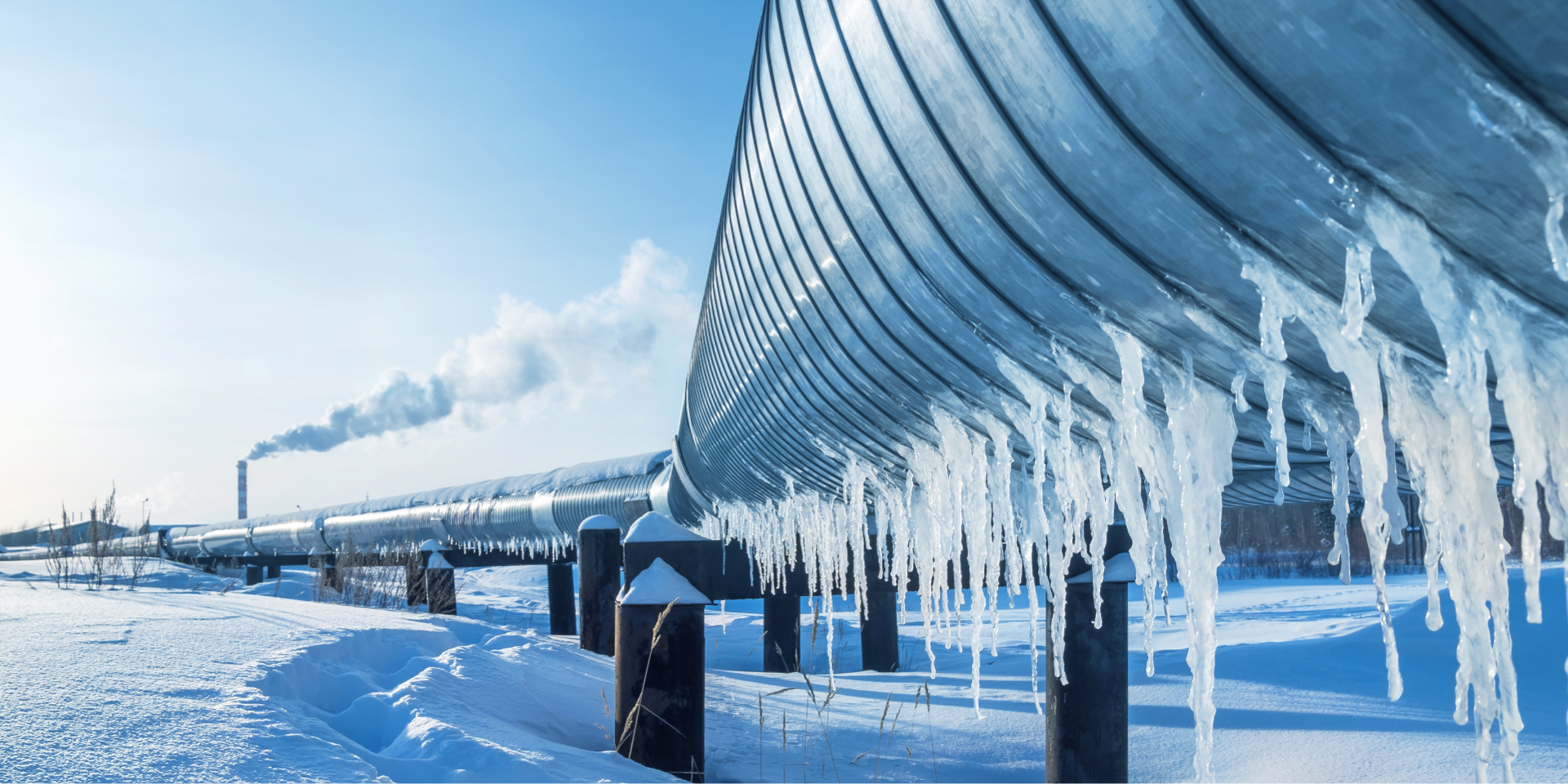
Throughout 2022, the reduction in Russian gas imports to the EU and the resilience of European energy markets have been subject of significant public discourse and policy-making. Of particular concern has been the EU’s ability to maintain its environmental goals, as substitution from Russian pipeline gas to liquified natural gas and other fuels such as coal, could result in increased emissions. This brief aims to reevaluate the consequences from the loss of Russian gas and the EU’s response to it on greenhouse gas emissions in the region. Our analysis suggests that the energy crisis did not result in a rise in emissions in 2022. While some of the factors that contributed to this outcome – such as a mild winter – may have been coincidental, the adjustments caused by the 2022 gas squeeze are likely to support rather than jeopardize the EU’s green transition.
Energy markets in Europe experienced a tumultuous 2022, with the Russian squeeze on natural gas exported to the region bringing a major shock to its energy supply. Much attention has been devoted to the effects of the succeeding spiking and highly fluctuating energy prices on households’ budget and on the production sector, with numerous policy initiatives aimed at mitigating these effects (see, e.g., Reuters or Sgaravatti et al., 2021). Another widely discussed concern has revolved the consequences of the gas crisis – such as switching to coal – on the EU’s climate policy objectives (see e.g. Bloomberg or Financial Times). In this brief, we analyze and discuss to what extent this concern turned out to be valid, now that 2022 has come to an end.
We consider greenhouse gas (GHG) emissions stemming from the main strategies that allowed the EU to weather the gas crisis throughout 2022 – namely the substitution from Russian gas to other energy sources. These strategies include increased imports of liquified natural gas (LNG), a lower gas demand, and an increased reliance on coal, oil, and other energy sources. We also discuss the implications of the crisis for climate mitigation in the EU and try to draw lessons for the future.
Substitution to LNG and Pipeline Gas from Other Suppliers
Prior to 2022, Russian natural gas largely reached Europe by pipeline (92.4 percent in 2021 according to Eurostat). More than half of these pipeline imports, 86 billion cubic meters (bcm), were lost during the 2022 Russian gas supply squeeze, predominantly through the shut-down of both the Yamal and the Nordstream pipelines. 57 percent of this “missing” supply was met through an increase in LNG imports from several countries, the largest contributor being the U.S. Another 27 percent of the “missing Russian gas” was substituted by an increase in pipeline gas imports from other suppliers, with the UK (20 percent) and Norway (7 percent) taking the lead. A substantial part of the replaced gas was stored, rather than combusted. With this in mind, here we concentrate on the upstream emissions associated with this change – i.e., emissions that occurred during the extraction, processing, and transportation. The change in the combustion emissions is postponed to the next section.
There is an ongoing debate in the literature on whether the greenhouse gas pollution intensity of LNG is higher or lower than that of gas delivered through pipelines – prior to final use. In comparison to pipeline gas, LNG is associated with emissions resulting from energy-intensive liquefaction and regassification processes in upstream operations as well as with fuel combustion from transportation on ocean tankers. For both LNG and pipeline gas it is also crucial to consider fugitive methane emissions, as methane has up to 87 times greater global warming potential than carbon dioxide in the first 20 years after emission, and up to 36 times greater in the first 100 years. One source of methane emissions is leaks from the natural gas industry (both “intentional” and accidental) since methane is the primary component of natural gas. Both LNG and pipeline gas infrastructure are subject to such leaks, and the size and frequency of these leaks during transportation varies greatly depending on the technologies used, age of infrastructure, etc. Further, the risk of these leaks may also be different depending on the technology of gas extraction.
Currently there is limited knowledge about the size of greenhouse gas emissions, including methane emissions resulting from leaks, from specific gas projects. Until recently, most estimates were based partially on self-reported data and partially on “emission factors” data. Modern and more reliable methods, for instance satellite-based measures for methane emissions, suggest that the resulting figures are greatly underestimated (see, e.g., Stern, 2022; IEA; ESA) but the coverage of these new estimates is currently limited.
As a result, there is considerable disagreement in the literature on the emissions arising from Russian pipeline gas imports vs. LNG imports to the EU. For example, Rystad (2022) argues that the average LNG imports to Europe have a CO2 emission intensity that is more than 2.5 times higher than that from pipeline gas from Russia (although they do not explicitly state whether these figures include fugitive methane emissions). On the contrary, Roman-White et al. (2019) suggest that the life-cycle GHG emission intensity of EU LNG imports (from New Orleans) is lower than EU gas imports from Russia (via the Yamal pipeline).
For the purposes of this exercise, we choose to rely on middle-ground estimates by DBI and Sphera, which assess GHG emission intensity along different Russian gas import routes (DBI, 2016) and across different LNG suppliers to the EU (Thinkstep – Sphera, 2020). This allows us to account for substantial heterogeneity across routes.
We also account for the change in upstream emissions associated with the switch from imports of pipeline Russian gas to pipeline gas imports from Norway and the UK. For this, we approximate the GHG emission intensity of the new flows using the estimate suggested by Thinkstep – Sphera (2017).
The results of our assessment are presented in the top three rows of Table 1. They suggest that a substitution from Russian gas imports to LNG imports and pipeline imports from other sources resulted in an increase in upstream GHG emissions by approximately 14 million tons (Mt) of CO2eq. Details on calculations and assumptions are found in the online Appendix.
Table 1. Change in EU GHG emissions resulting from Russian gas squeeze.

Source: Authors’ own calculations based on DBI (2016), McWilliams et al. (2023), Sphera (2017; 2020), and IEA (2022). See the online Appendix for more details on assumptions, calculations, and sources. Note: Billion cubic meters (bcm) and terawatt-hours (TWh).
The Decline in Gas Demand and the Switch to Other Fuels
A decrease in gas use in the EU constituted another response to the Russian gas squeeze. Gas demand in the EU is estimated to have declined by 10 percent (50 bcm or 500 TWh) in 2022 with respect to 2021 (IEA, 2022). Part of this decline was facilitated by switching from gas to other polluting fuels, such as oil and coal. The extent to which switching occurred however differed across the three main uses of gas; power generation, industrial production, and residential and commercial use. Below we discuss them separately.
Power Generation
At the onset of the 2022 energy crisis, a prevalent expectation was that there would be significant gas-to-coal switching in power generation. However, gas demand for power generation, which accounts for 31.4 percent of the gas demand from EU countries (European Council), increased by only 0.8 percent in 2022 (EMBER, 2022, p.29), implying that there was no direct substitution from gas-fired to coal-fired generation.
One of the reasons to why there was no major switching to coal in spite of the increase in gas prices is that CO2 emissions are priced in the Emissions Trading System (ETS) program, and the average carbon price has been growing recently, reaching an average of around €80/ton in 2022. Given that coal has a higher emission intensity than gas, the carbon price increases the relative cost of coal versus gas for power generators.
Instead, the decline in demand came from industry, residential and commercial use, which together account for nearly 57 percent of the EU’s gas demand (European Council).
Industry Use
For the industry, IEA calculations (2022) suggest a demand drop of 25 bcm, which would correspond to approximately 50 Mt CO2eq. However, half of the industrial gas reduction came from gas to oil switching. Based on our estimates, this switch implies an additional 41 Mt CO2eq emissions, considering both upstream emissions and emissions from use in furnaces (assuming this to be the prevalent use of the oil that substituted gas, see McWilliams et al., 2023). The remaining half of the industrial demand decline resulted from energy-efficiency improvements, lower output, and import of gas-intensive inputs where possible (ibid.). These changes are either neutral in terms of life-cycle emission impact (import increases) or emission-reducing (efficiency improvements and lower output).
Residential and Commercial Use
Residential and commercial use represented the remaining part of the 500 TWh gas demand decline. In this case, lower gas demand is unlikely to imply massive fuel switching to other fossil fuels, simply because of the lack of short-term alternatives. For example, European households use gas mostly for space heating and cooking, and albeit both higher use of coal for home-heating (BBC) and a surge in installations of heat pumps (Bruegel, 2023 and EMBER, 2023) have been reported, the net change in emissions resulting from these two opposite developments is likely relatively minor as compared to other considered sizeable changes.
The Rise of Coal
As observed, there was no direct switch from gas to coal in European power generation. However, coal generation in the EU did increase by 6 percent in 2023 (IEA, 2022), to help close the gap in electricity supply created by the temporary shut-down of nuclear plants in France and the reduced performance of hydro. In our calculations we assume that in a counterfactual world with no Russian gas squeeze, gas-fired electricity would have covered most of the gap that was instead covered by coal. Therefore, we estimate that, as an indirect result of the Russian gas squeeze in 2022, CO2eq emissions increased by 27 Mt, specifically because of the ramp-up in coal generation (see the second section in Table 1).
Gas Shortage and the EU’s Climate Objectives
In recent years, the EU has made substantial progress in climate change mitigation. Despite widely expressed concerns, it achieved its 2020 targets – reducing emission by 20 percent by 2020, from the 1990 level. However, its current target of a 55 percent net GHG emission reduction by 2030, requires average yearly cuts of 134 Mt CO2eq, from the 2021 level. This is an ambitious target: while the emission cut between 2018 and 2019 exceeded this level, the average yearly cut between 2018 and 2021 however fell short (Eurostat).
The question is if the Russian gas squeeze can significantly undermine the EU’s ability to achieve these climate goals?
First, based on our assessment above, the changes prompted by the Russian squeeze – namely a move from pipeline-gas to LNG, a decline in gas demand and an increase in coal and oil use – made 2022 emissions decline by 18 Mt CO2eq. This suggests that the energy shock prompted overall emission-reducing adjustments in the short run. One important question that arises from this is therefore how permanent these adjustments are.
The increased reliance on LNG (and other gas suppliers) is likely to be permanent as a return to imports from Russia is hardly imaginable and as the 2022 surge in LNG imports entailed significant investments and contractual obligations. According to our estimates, overall, this shift is going to cause a relatively modest increase in yearly CO2eq emissions, approximately 10 percent of the needed emission reduction outlined above. Moreover, this is accounting for emissions throughout the EU’s entire supply chain – which is increasingly advocated for, but not currently applied in the typical emission accounting. It is, of course, important to make sure that ongoing LNG investments do not result in “carbon lock-ins”, postponing the green transition.
The decline in gas demand is a welcome development for climate mitigation if it is permanent. Part of the decline, from improved energy efficiency or installation of heat pumps, is indeed permanent. However, European households also responded temporarily (to warmer than usual winter and high gas prices (for instance by reducing their thermostats). Their behavior in the near future will therefore depend on the development of both these variables.
Overall, our assessment is that the Russian gas squeeze did force some adjustments in demand that might translate into a permanent decline in greenhouse gas emissions.
The question however remains of how the shortage of gas can be met in a scenario with higher gas demand due to, for instance, colder winters. In terms of climate objectives, it is of paramount importance that coal-powered generation does not increase (which would happen if, for instance, the price of gas continues to raise due to shortages). In this sense some lessons can be learned from the response to the shortage in electricity supply following the exceptional under-performance of nuclear and hydro in 2022. Wind and solar, which provide the lowest-cost source of new electricity production, in combination with declines in electricity demand, were able to cover 5/6 of the 2022 shortage created by the nuclear and hydro shock (EMBER, 2023), thus relegating coal to a residual contribution. We expect this pattern to emerge also in the future in the presence of other crises. However, we also caution that the lower production of electricity was at least partially caused by the dramatic heatwaves and droughts experienced throughout the summer in Europe. These events are likely to happen more often in the face of climate change. European policy-makers should therefore carefully assess the capacity of the EU energy system to address potentially multiple and frequent shocks with minimal to no-reliance on coal, in a scenario where also reliance on gas needs to be in constant decline given the Russian gas squeeze and unreliability.
Finally, the dramatic circumstances of 2022 led the EU to adopt the REPowerEU plan, which outlines financial and legal measures to, among other things, speed up the development of renewable energy projects and induce energy-saving behavior.
The outlined observations lead us to conclude that the Russian gas squeeze is ultimately unlikely to sizably reduce the chances of the EU reaching its climate goals, suggesting that the 2022 concerns in this regard were somewhat exaggerated. Nonetheless, learning from the costly lessons of the 2022 energy crisis is crucial for efficient policy making in the future.
References
- BBC. (2022, September 29). Energy prices: Households turning to coal ahead of ‘hard winter’. https://www.bbc.com/news/uk-england-somerset-63072561
- Bloomberg. (2022, July 22). Putin’s War Threatens Europe’s Ambitious Climate Goals. https://www.bloomberg.com/news/articles/2022-07-07/ukraine-invasion-threatens-europe-s-climate-change-goals#xj4y7vzkg
- DBI. (2016). Critical Evaluation of Default Values for the GHG Emissions of the Natural Gas Supply Chain. https://www.dbi-gut.de/emissions.html?file=files/PDFs/Emissionen/Report_english.pdf&cid=5808
- EMBER. (2023). European Electricity Review 2023. https://ember-climate.org/insights/research/european-electricity-review-2023/#supporting-material-downloads
- ESA. (2020). Mapping methane emissions on a global scale. https://www.esa.int/Applications/Observing_the_Earth/Copernicus/Sentinel-5P/Mapping_methane_emissions_on_a_global_scale
- European Commission. (2022). REPowerEU: affordable, secure and sustainable energy for Europé. https://commission.europa.eu/strategy-and-policy/priorities-2019-2024/european-green-deal/repowereu-affordable-secure-and-sustainable-energy-europe_en
- European Council. (2023). Infographic – Where does the EU’s gas come from? https://www.consilium.europa.eu/en/infographics/eu-gas-supply/#:~:text=Gas%2520consumption%2520in%
2520the%2520EU&text=Over%252030%2525%2520is%2520used%2520for,
accounts%2520for%2520just%2520over%252011%2525 - Eurostat. (2023). https://ec.europa.eu/eurostat/databrowser/view/ENV_AC_AIGG_Q/default/table?lang=en&category=env.env_air.env_air_aa
- Financial Times. (2020, June 20). EU Warns against Fossil Fuel ‘Backsliding’ as Coal Replaces Russian Gas. https://www.ft.com/content/a8b179e2-b565-42b6-bb41-90aea44536e1.
- ICAP. (2023). ICAP Allowance Price Explorer. https://icapcarbonaction.com/en/ets-prices
- IEA. (2020). Global methane emissions from oil and gas. https://www.iea.org/articles/global-methane-emissions-from-oil-and-gas
- IEA. (2022). “How to Avoid Gas Shortages in the European Union in 2023”, https://www.iea.org/reports/how-to-avoid-gas-shortages-in-the-european-union-in-2023
- IEA. (2023). Electricity Market Report 2023. https://www.iea.org/reports/electricity-market-report-2023
- McWilliams, B., Sgaravatti, G. and Zachmann, G. (2021). European natural gas imports. Bruegel Datasets, first published 29 October, available at https://www.bruegel.org/publications/datasets/european-natural-gas-imports/
- McWilliams, B., Tagliapietra, S., Zachmann, G. and Deschuyteneer. T. (2023). Preparing for the next winter: Europe’s gas outlook for 2023. Policy Brief 01/2023, Bruegel. https://www.bruegel.org/policy-brief/european-union-gas-survival-plan-2023
- Reuters. (2023, February 13). Europe’s spend on energy crisis nears 800 billion euros. https://www.reuters.com/business/energy/europes-spend-energy-crisis-nears-800-billion-euros-2023-02-13/
- Roman-White, S., Rai, S., Littlefield, J., Cooney, G. and Skone, T. J. (2019). Life cycle greenhouse gas perspective on exporting liquefied natural gas from the United States: 2019 update. DOE/NETL-2019/2041. National Energy Technology Laboratory. https://www.energy.gov/sites/prod/files/2019/09/f66/2019%20NETL%20LCA-GHG%20Report.pdf
- Rystad. (2022). LNG import boom could drive up European emissions by 35 million tonnes. Rystad Energy.
- Sgaravatti, G., Tagliapietra, S., Trasi, C. and Zachmann, G. (2021). National policies to shield consumers from rising energy prices. Bruegel Datasets. https://www.bruegel.org/dataset/national-policies-shield-consumers-rising-energy-prices
- Thinkstep – Sphera. (2017). Greenhouse Gas Intensity of Natural Gas. http://gasnam.es/wp-content/uploads/2017/11/NGVA-thinkstep_GHG_Intensity_of_NG_Final_Report_v1.0.pdf
- Thinkstep – Sphera. (2020). Life Cycle Emissions of Natural Gas Transported via TurkStream. https://energijabalkana.net/wp-content/uploads/2021/10/ts-Sphera-LCA-TurkStream_Final-Report.pdf
- Stern, J. P. (2022). Measurement, Reporting, and Verification of Methane Emissions from Natural Gas and LNG trade: Creating transparent and credible frameworks, OIES Paper: ET, No. 06, ISBN 978-1-78467-191-4, The Oxford Institute for Energy Studies, Oxford.
Online Appendix
Disclaimer: Opinions expressed in policy briefs and other publications are those of the authors; they do not necessarily reflect those of the FREE Network and its research institutes.
Will New Technologies Change the Energy Markets?

With an increasing world demand for energy and a growing pressure to reduce carbon emissions to slow down global warming, there is a growing necessity to develop new technologies that would help addressing demand and carbon footprint issues. However, taking into account the world’s dependence on hydrocarbons the question remains – can new technologies actually change the energy markets? In this policy brief, we highlight challenges and opportunities that new technologies will bring for energy markets, in particular wind energy, smart grid technology, and electromobility, that were discussed during the 10th SITE Energy Day, held at the Stockholm School of Economics on October 13, 2016.
The expanding world population and economic growth are considered the main drivers of the global energy demand. Up to 2040, total energy use is estimated to grow by 71% in developing countries and by 18% in the more mature energy-consuming OECD economies (IEA, 2016). In parallel, many countries (including the world’s biggest economies and largest emitters: USA and China) have signed the Paris agreement – the first-ever universal, legally binding global climate deal that aims to reduce emissions and to keep the increase in global average temperature from exceeding 2°C above pre-industrial levels.
Meeting a growing global energy demand, and at the same time reducing CO2 emissions, cannot be achieved by practicing ‘business as usual’. It will require some fundamental changes in the way economic activity is organized. In this context, the development of new technologies and how it will affect the energy sector is a crucial element.
Wind power, smart grid, and electromobility
With technological progress and support schemes to decrease CO2 emissions, wind energy is now a credible and competing alternative to energy produced from coal, gas and oil. In 2015, wind accounted for 44% of all new power installations in the 28 EU member states, covering 11.4% of Europe’s electricity needs (see here).
This new technology has triggered a downward pressure on energy prices because of a “Merit order effect” (i.e. a displacement of expensive generation with cheaper wind). While consumers may appreciate this development, Ewa Lazarczyk Carlson, Assistant professor at the Reykjavik University (School of Business) and IFN, stressed that the increasing importance of wind energy challenges the functioning of electricity exchange. First, a lower price has reduced the incentives to invest in conventional power plants necessary when the wind is not blowing or when it is dark. Moreover, with the renewable energy intermittency, the probability of system imbalance and price volatility has increased. In turn, this has led to an increase of maintenance costs for conventional generators due to their dynamic generation costs (i.e. start-ups and shut-down costs).
Digital technology has gradually been used in the energy sector during the last decades, changing the way energy is produced and distributed. With smart grid (i.e. an electricity distribution system that uses digital information) energy companies can price their products based on real time costs while customers have access to better information, allowing them to optimize their energy consumptions. Sergey Syntulskiy, Visiting Professor at the New Economic School in Moscow, stressed that smart grids have had at least two effects. They have made the integration of renewable energy to the system easier and have allowed for prosumers, i.e. entities that both consume and produce energy. The next step is to develop new regulatory incentives to optimize energy systems as well as to provide a legal framework for the exchange of information in the energy sector.
One of the main pollutants has long been the transport sector that accounts for 26% energy-related of CO2 emission (IEA, 2016). Electromobility – that is, use of electric vehicles – is often considered the solution for this problem. When this technology is widely adopted, a major switch from oil to electricity is expected for the transportation sector. Mattias Goldmann, CEO of Fores, argued that even if electromobility will improve air quality and reduce noise levels in cities, its positive impact relies on smart grids and locally produced energy. Moreover, the environmental benefits will be ensured only if electric energy is produced from renewable and clean sources.
Toward a carbon-neutral energy system?
The Nordic countries are currently pushing for a near carbon-neutral energy system in 2050. Markus Wråke, CEO at the Swedish Energy Research Centre, emphasized that the Nordic Carbon-Neutral Scenario is only feasible if new technologies allow for a significant change of energy sources and a better interconnected market (see report by IEA 2016 b).
To cut emissions, a decrease in oil and gas consumption in energy production and within the transport sector is needed (see Figure 1). The adoption of electric vehicles (EVs) and hybrid cars is very likely to drastically increase in the next decades (EVs may have a share of 60% of the passenger vehicle stock in 2050, IEA 2016b).
Figure 1. Nordic CO2 emissions in the CNS
There are currently limited technology options to reduce emissions for big industrial energy consumers. Moreover, there is a concern that those industries may choose to relocate if the Nordic emission standards are too strict. It is therefore important to have low and stable electricity prices. This can only be achieved if cross-border exchanges are improved (which means that the electricity trade in the Nordic region will have to increase 4-5 times by 2050). It is unclear however how policy makers will create a regulation that incentivizes energy companies to build interconnections and increase trade both between the Nordic countries, and the Western and Eastern European countries.
Figure 2. Electricity trade 2015 and 2050
Energy producers
Another concern is that energy-exporting and energy-importing countries may have opposing attitudes towards investing and developing new energy technologies. Countries among the biggest energy producers and exporters depend on a stable demand and price for energy. For example, Russian GDP growth depends between 50-92% on the oil price, depending on the variables used for calculations, as mentioned by Torbjörn Becker, Director of SITE. For large exporters of hydrocarbon, new energy technologies may be seen as a threat because of a potentially reduced energy demand and an increased price volatility that will, in turn, create fundamental issues to balance state budgets and improve living standards.
Figure 3. The Relationship between Russian GDP and oil price
Source: Calculations by Torbjörn Becker, October 13, 2016
The challenge of security of supply
To summarize, new energy technologies will drive energy companies towards optimizations and cost cutting, bring previously unseen connectivity to energy markets and make energy markets more complex. Samuel Ciszuk, Principal Advisor at the Swedish Energy Agency, stressed that interconnected, more complex and interdependent energy systems might increase the vulnerability of energy systems to external threats and intimidates to decrease the security of supply. Technological change and increased competition with lower profit margins will force companies to minimize their expenditure on energy production, storage and transmission and to find cheaper financing options. Optimization and searches for cheaper financing instruments will push energy companies towards selling some of the company assets to financial investors. These changes will create a more decentralized energy market, with more players. Such energy systems will become harder to govern in times of an energy crisis and external threats. Policy makers will have to design new and more complex regulations to fit the needs of the transforming energy markets.
References
- Fogelberg, Sara and Ewa Lazarczyk, 2015. “Wind Power Volatility and the Impact on Failure Rates in the Nordic Electricity Market”, IFN Working Paper 1065.
- IEA, Annual Energy Outlook, 2016a.
- IEA/OECD/Norden, 2016b. “Nordic Energy Technology Perspectives” (see here)
- Speaker presentation from the 10th Energy day, 2016 (see here)